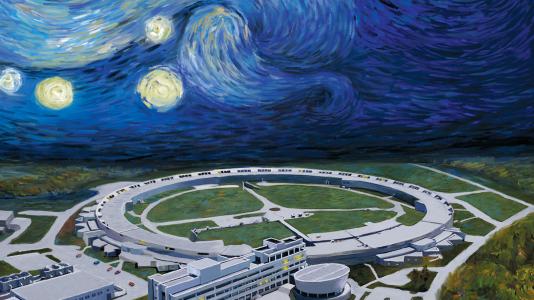
In a few short hours, she’ll have exhausted a 36-hour window she’s earned to get a glimpse into the early days of the planet using one of America’s most advanced scientific tools.
Brounce and her colleague, Mary Peterson, have collected tiny chips of glass formed by volcanic eruptions all over the world and brought them to Argonne’s Advanced Photon Source, known to its users as the APS. Here at Sector 13, they’ll use powerful X-rays from the APS to unlock the secrets of each volcano’s elemental composition.
Right now, as the X-ray beam scans a chip of a 61-million-year-old volcano from Baffin Island in Canada, Brounce and Peterson have been awake—save for quick naps—for almost a full day. But the APS is, by necessity, indifferent to the circadian rhythms of its users. To squeeze as much scientific discovery as possible out of every minute, the APS runs around the clock, 24 hours a day, with only scheduled breaks for maintenance every few months.
For the geologists, access to such a unique facility is worth the sacrifice of sleep. “I feel like you can do anything for 48 hours,” Brounce says.
Before the two scientists traveled here, Brounce from Caltech and Peterson from the University of New Hampshire, they spent weeks assembling a collection of glass from colleagues studying volcanoes from Madagascar to Guam to Iceland. Then they carefully shaved and polished each piece down, like a jeweler might, so that each chip is no more than 150 microns thick—about the width of a single human hair.
During their remaining few hours at the beam, they will use two sets of tweezers to position each chip in the path of the beam, where the APS’s intensely bright X-rays will strike it. Each element in the chip will absorb the X-rays slightly differently and re-emit them at a specific energy. This will tell Brounce and Peterson the exact elemental composition of the glass. Armed with this information from chips from volcanoes around the world, they can infer whether the young Earth’s atmosphere and rocks were oxygen rich or not, and how this changed as the world aged.
Researchers like volcanoes because they bring up ancient rock from deep below the surface. “The Baffin Island rock could turn out to have very primitive signatures,” says Brounce. “Perhaps even a record of the formation of the Earth; that is, 4 billion years ago.”
The Advanced Photon Source is a giant ring, two-thirds of a mile in circumference, in which electrons circle at nearly the speed of light. Its job is to make very powerful X-rays, which it does by accelerating the electrons and then bending their paths with magnets. This throws off energy in the form of X-rays.
These X-rays are enormously useful to scientists who want to see the chemical and molecular structures of objects. Unlike researchers at accelerators like the Large Hadron Collider, who are looking to understand the fundamental particles that make up the universe, scientists use the APS like a giant microscope to look at the materials things are made of: solar cells, cast iron, spider silk, batteries, even the paint from famous paintings. (A few days after Brounce and Peterson leave a team will be at Sector 13 to study the composition of the paint in Edward Munch’s painting The Scream.)
There are 35 different “sectors” where X-rays peel off from the ring, so at any given moment the APS actually houses dozens of different experiments conducted by scientists from all over the world under one roof.
Fortunately for Brounce and Peterson, some parts of their experiment can be monitored remotely, from a little hotel built near the APS expressly to house researchers in the midst of round-the-clock studies.
“We’ll go back to the hotel and catch a little sleep in a few hours,” says Peterson, surrounded by coffee cups. “But we do set an alarm every hour just to wake up and check the feed to see that it’s all going smoothly. You don’t want to have something go wrong and then you’re sleeping away your beam time.”
The Sector 13 beamline is part of the center for Advanced Radiation Sources at the University of Chicago.
Each sector has its own group of resident beamline scientists who assist visiting scientists with their runs, work on equipment and detectors to improve performance, and do their own research. When Brounce and Peterson had a hardware issue at about 8 p.m. that they couldn’t fix, they called Matt Newville, a Sector 13 beamline scientist. He was home for the day, but he went to his computer at home, connected to the beamline, and made a tiny adjustment to the beam’s trajectory that fixed the problem.
In all, Brounce and Peterson will test 50 to 75 samples from different volcanoes during their allotted beam time. Once they have their data, they’ll take it back to their home universities to dissect all the information they’ve just collected, and see what they can tease out about the beginning of the planet.
On the other side of the ring, in Sector 34, two other scientists are tapping the same flow of X-rays. But Brian May and Mark Wolf, both from the University of Illinois at Chicago, are using their X-rays to look at batteries: specifically, lithium-ion batteries, like the kind in your cell phone or laptop.
Scientists are racing to find new ways to make better lithium-ion batteries because in today’s society their progress is inextricably linked to leaps forward in technology (can we power cars for 500 miles?) as well as electricity and sustainability (can we make a battery that can store enough energy to make widespread wind and solar power more realistic?).
Batteries, however, are a complex bit of technology. When you charge and then use your battery, you are shuttling lithium ions back and forth between the two terminals of the battery. Inevitably, as with all moving things, this wears out the physical structure of the battery. May and Wolf are using X-rays to see exactly how that structure breaks down over time.
“Our group has been here many times,” May says. “We learn something new every time.”
May and Wolf have worked on several different beamlines. Each beamline has specialized equipment designed to tackle specific scientific questions. Some are set up to take very precise readings over a small area; others scan a larger area more quickly. Some specialize in geology; others examine frozen proteins, or objects under high pressure.
And some are designed to take readings as a battery is running—that’s the one May and Wolf are using right now. This beamline gives them information about the atomic structure of the battery; they’ll combine it with data from another beamline to get chemical information as well, for a more complete picture.
May and Wolf also experimenting with a new technique to create a 3-D reconstruction. If they can watch how the battery’s structure breaks down over time, they can figure out how to build a more resilient one.
Their experience on the beam also means they’ve had time to refine their technique for keeping their focus late into the night.
“I budget about a case of Mountain Dew per run,” May says.
“Sometimes to stay awake, I’ll go for a ride on the trikes, just to get the blood flowing,” Wolf says. (There are big three-wheeled bikes scattered around the ring for people who have to travel far around the experiment hall.)
“I like it. I get in my zone late at night,” May says.
At 8 a.m. May and Wolf’s run ends, and they collect their battery samples and data. More teams are waiting right behind them, ready to begin their shifts at the beam.
But there’s another group working at the APS who stay as the others come and go. They’re the people that keep the APS’s brain working and blood flowing, and that means they keep watch 24 hours a day, every day.
The highest priority for the dozens of people who run the APS is keeping interruptions to the X-ray beam at an absolute minimum. The APS is so large that it has its own utility plant, a large hall beating with water pumps and deionizers, air compressors, electrical equipment, and emergency backup generators. “If the APS experiment hall is the body, this is its heart,” says Argonne maintenance mechanic Dave Kazenko, and it’s his and his colleagues’ job to keep it pumping.
On the floor of the ring, engineer Glenn Kailus’ maintenance team walks the experimental hall night and day, checking for water leaks and responding to emergencies: everything from disconnecting the power locally so that a scientist can tweak the equipment (usually a few times a day) to leaks, malfunctions, or a bat loose in the lobby (Argonne is surrounded on all sides by a nature preserve). “No two days are ever the same here,” Kailus says.
Even though the APS hosts thousands of users and visitors every year, it still has its very occasional lonely moments for those to whom the ring is most familiar. “It can get a little weird when we’re in a maintenance shutdown and you’re maybe the only person in a million square feet of space,” says maintenance engineer Roger Camacho.
The beam pauses only for scheduled maintenance. When President Obama visited the APS to hold a press conference in 2013, and before him President Bush in 2002, the APS crew kept the beam running as usual. Science stops for no one, not even the president of the United States.
It barely even stops for a natural disaster.
“Something like an earthquake, we can deal with that easy,” says Randy Flood, who heads the APS control room.
When a magnitude-7.6 earthquake shook the ground in Peru last year, the vibrations traveled thousands of miles across the world and threw the APS’s exquisitely sensitive beam off by a few microns. It shut itself down, sending dozens of alarms sounding in the control room.
It was less than 10 minutes before his team got the beam back online, Flood says. These earthquake pauses happen perhaps once every year or two. Beam pauses are more generally caused by routine equipment failures, but all are typically fixed in less than 30 minutes. He’s proud of his team, he says, as well as the other 450 employees at the APS, who work rotating shifts and miss holidays so that the beam is exactly where the users need it to be.
Thanks to all these people, the beam is running smoothly. One of its next stops around the ring is destined for the flash-frozen proteins of a bacterium called Stigmatella aurantiaca.
Depending on your perspective, S. aurantiaca may or may not be considered a beautiful organism—wherever it travels, it leaves copious trails of slime as it goes—but it is certainly a unique one. It forms odd fruiting bodies by means of the most complex behavior and life cycle of any known bacteria; and it lives on decaying organic matter, such as logs on a forest floor, eating both the rotting wood and the things that have come to eat the rotting wood.
Which is why it’s strange that it also carries genes for photoreceptors: proteins that react to light and dark, even though S. aurantiaca can’t photosynthesize food from light.
Emina Stojković, a professor at Northeastern Illinois University, wants to know why. This desire fits into a larger movement in the biology world. Researchers love photoreceptors, because they’re tailor-made bits of complex machinery that we could adapt for our own purposes. For example, scientists are looking into using bacterial photoreceptors as fluorescent markers, which “could revolutionize the way we visualize internal organs,” Stojković says.
But all this depends on having a complete understanding of how they work, which we don’t. Photoreceptors are actually made up of lots of proteins assembled together into an intricate machine, and no one has ever managed to collect a full picture.
Luckily, the APS has a storied history of helping researchers figure out the structures of proteins. Protein work here has led to drugs to combat HIV and several forms of cancer, as well as two Nobel Prizes for chemistry.
So Stojković and her students at the university have grown hundreds of petri dishes of S. aurantiaca under both light and dark conditions, then carefully isolated the photoreceptor proteins and crystallized them.
They bring the crystals here to Sector 19, carefully covered because they will shatter if exposed to light, and flash freeze them with liquid nitrogen in order to put them into the beam. Then, from what the X-rays reveal, they can piece together what the photoreceptors look like.
“It’s like a puzzle,” says Rima Rebiai, one of Stojković’s graduate students, and each crystallized protein they test gives them another tiny puzzle piece.
“The crystals are gorgeous. They look like emeralds,” says graduate student Trish Waltz, because you can only look at them under a green light so as not to shatter the crystals. “But they might have ice crystals in them.” Ice crystals interfere with the picture they’re trying to take of the structure, and they won’t know which crystals have ice until they get them in the beam. “Some of the ugliest crystals turn out to make the best diffractions,” she says, shrugging.
For Stojković, the APS is a comfortable and familiar place. She’s come to the APS almost as long as it’s existed: she first ran experiments here as a graduate student, and now, as a professor, she brings her own students. People still work here who worked here then, and they know her by name.
“I’ll continue using the APS for the rest of my career. It’s essential to my work,” she says. “It’s a wonderful resource that means so much to me and my students. And the staff here is amazing.”
Like the geologists and the battery researchers, Stojković’s experiment runs late into the night. She generally tries to spare her students the late hours, so for her, alone in the overnight periods, music is essential.
“You need something that changes the rhythm up, to stay awake,” she says. The sectors are far enough apart that you can play music without bothering the users next door. Sometimes, very late at night, Stojković says, you might catch her singing aloud to the protein crystals in the beam.
It’s a Friday night. Most of the population at Argonne, those with day jobs, have long since left—but the lights are still on at the Advanced Photon Source, and they will be all weekend, and the week after that, and the week after that. After all, Stojković’s shift ends in another 12 hours, and behind her is a team with a whole new set of questions waiting to be answered.
The Advanced Photon Source is a U.S. Department of Energy Office of Science User Facility. The GeoSoilEnviroCARS (GSECARS) national user facility manages the APS Sector 13 beamline for the University of Chicago; GSECARS, part of the Center for Advanced Radiation Sources at the University of Chicago, is supported by the National Science Foundation, Earth Sciences and the U.S. Department of Energy, GeoSciences. The Northeastern Collaborative Access Team (NE-CAT) facility is funded by the National Institute of General Medical Sciences from the National Institutes of Health; it is managed by Cornell University and consists of member institutions Columbia University, Cornell University, Harvard University, Memorial Sloan-Kettering Cancer Center, the Massachusetts Institute of Technology, Rockefeller University, and Yale University. Sector 19 is operated by Argonne’s Structural Biology Center, which is funded by the U.S. Department of Energy Office of Biological and Environmental Research. May and Wolf’s work is part of the NorthEast Center for Chemical Energy Storage (NECCES), an Energy Frontier Research Center funded by the U.S. Department of Energy.
Argonne National Laboratory seeks solutions to pressing national problems in science and technology. The nation’s first national laboratory, Argonne conducts leading-edge basic and applied scientific research in virtually every scientific discipline. Argonne researchers work closely with researchers from hundreds of companies, universities, and federal, state and municipal agencies to help them solve their specific problems, advance America’s scientific leadership and prepare the nation for a better future. With employees from more than 60 nations, Argonne is managed by UChicago Argonne, LLC for the U.S. Department of Energy’s Office of Science.
The U.S. Department of Energy’s Office of Science is the single largest supporter of basic research in the physical sciences in the United States and is working to address some of the most pressing challenges of our time. For more information, visit the Office of Science website.
All-nighters for Science
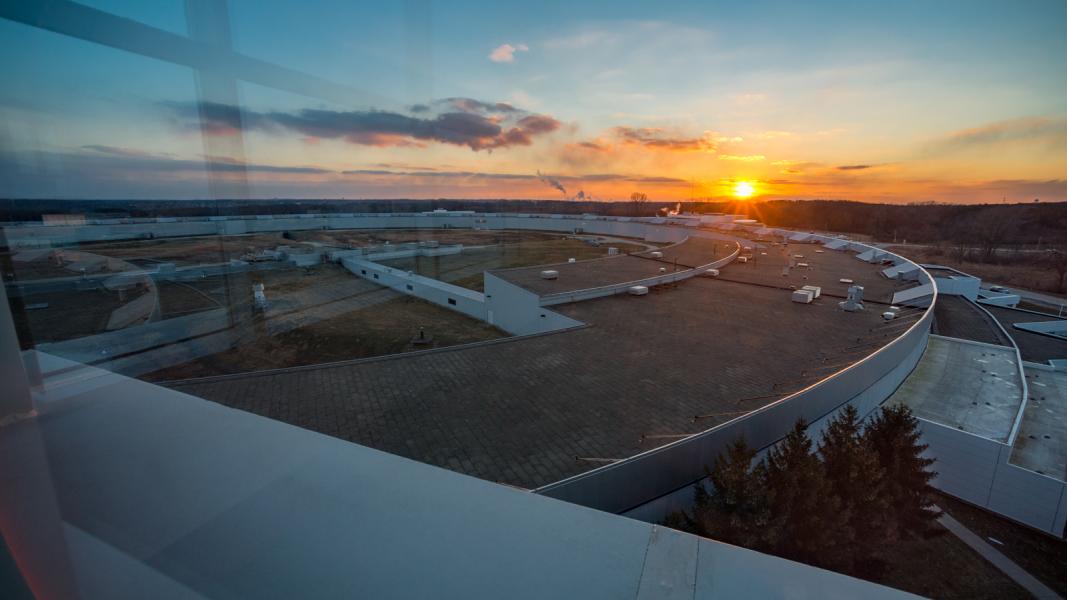
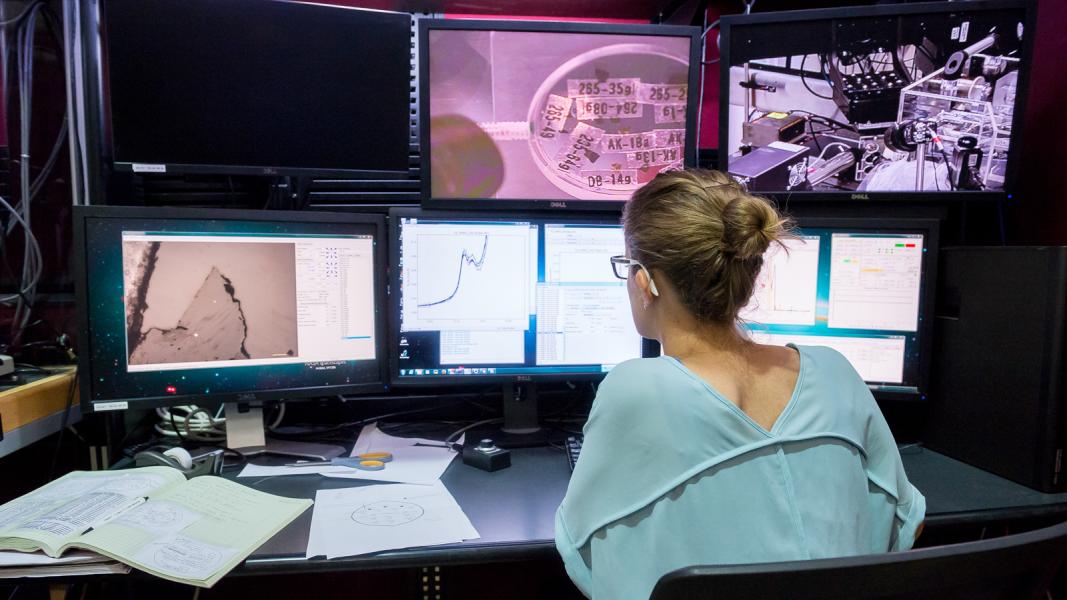
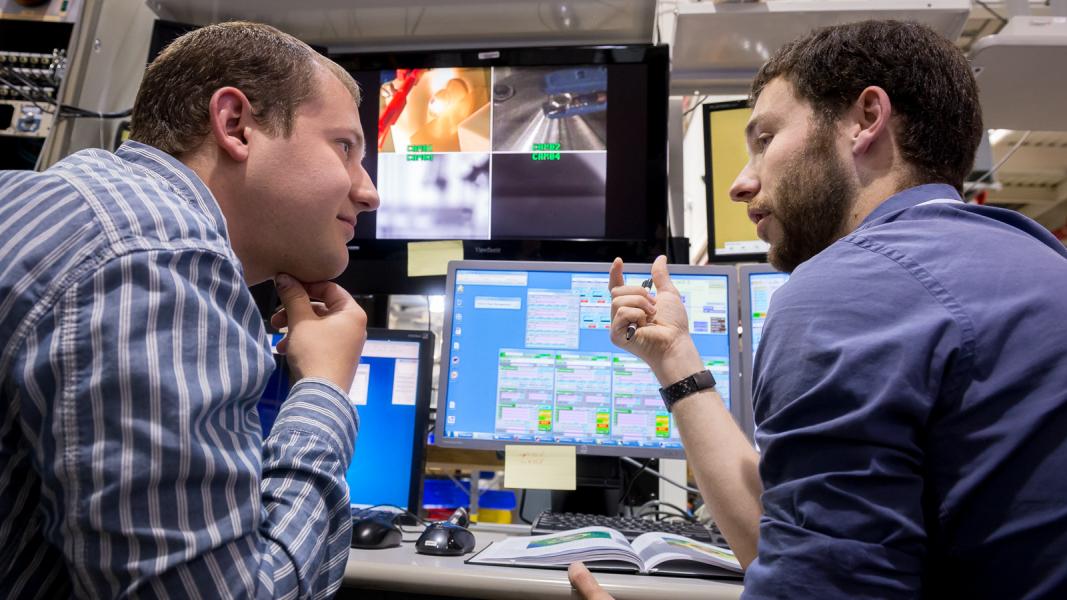
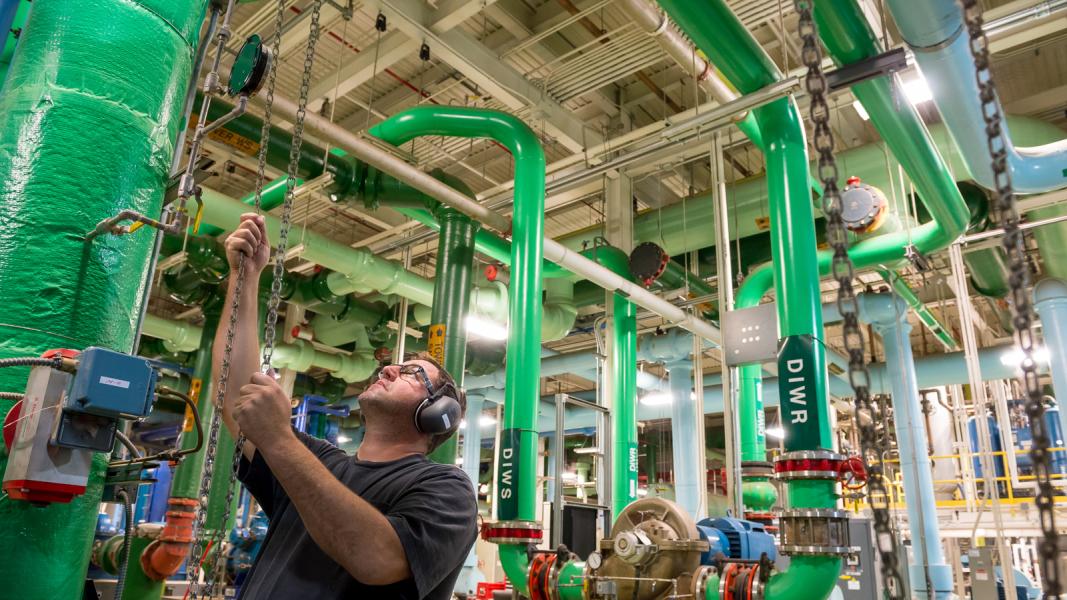
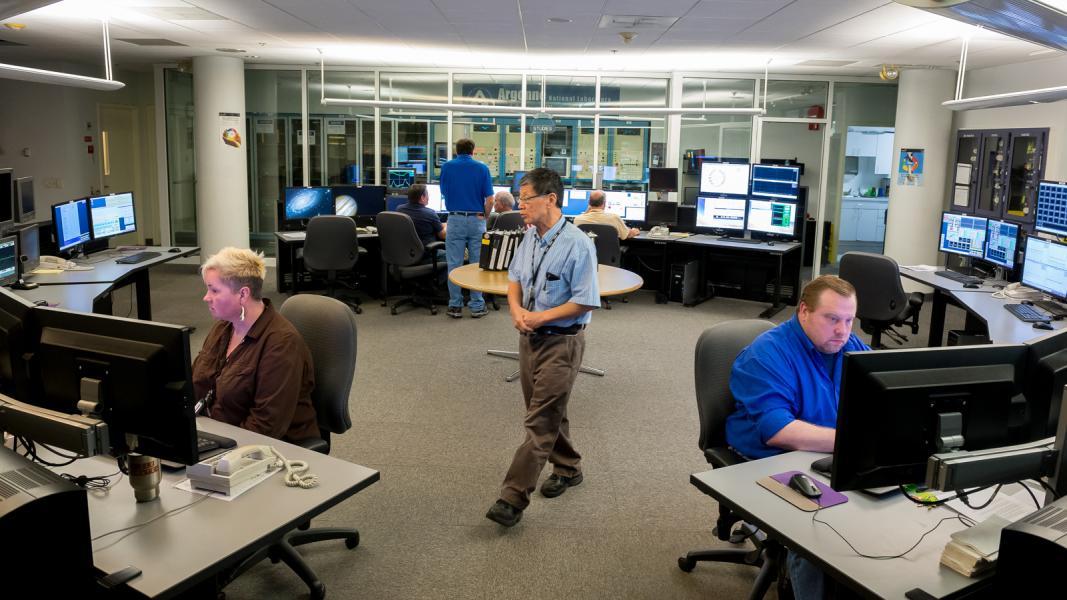
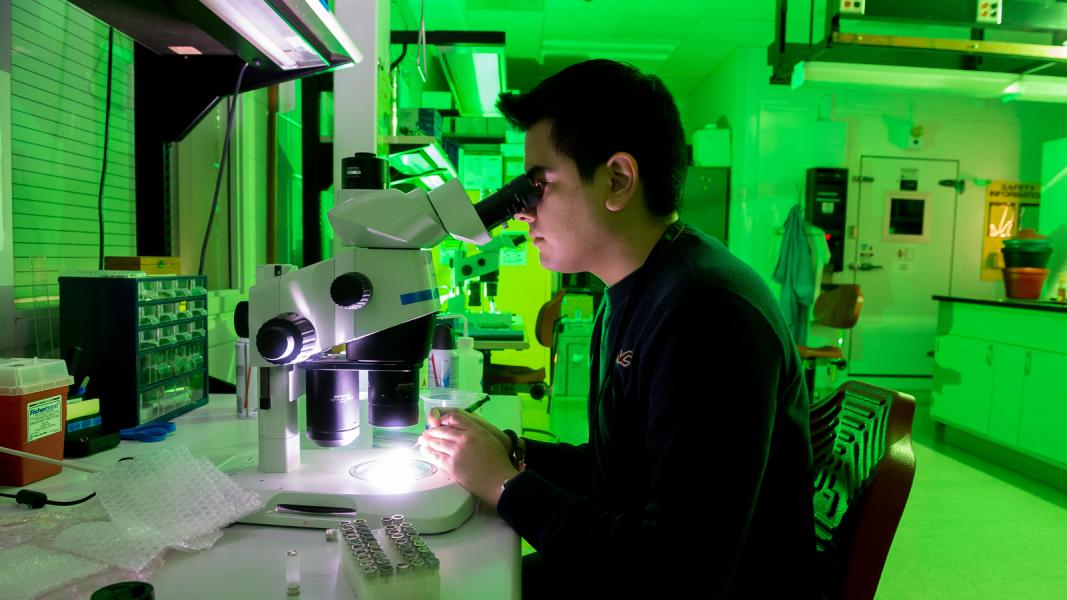
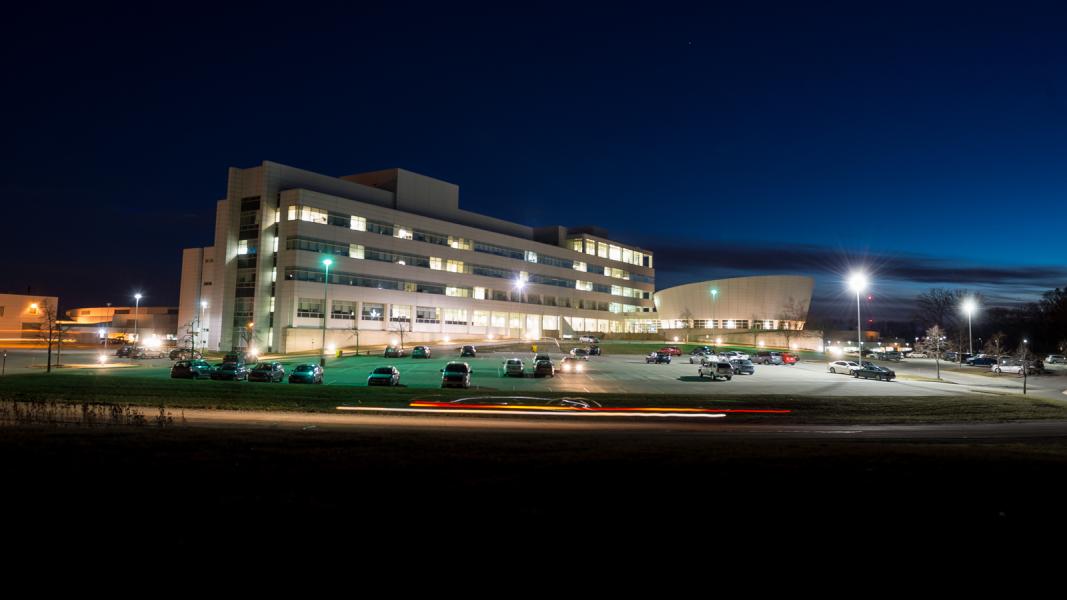