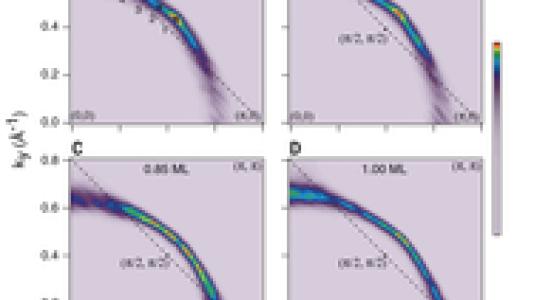
Researchers at the U.S. Department of Energy’s Argonne National Laboratory found that a class of materials called iridate oxides expresses characteristics that may shed light on the physics underlying an important class of superconductors.
The discovery, published in yesterday’s issue of Science, advances fundamental understanding of ingredients thought critical to high-temperature superconductivity and could someday help expand the list of known superconductors.
Superconductors are so named because they can conduct electricity with virtually zero loss to resistance. Their enormous technological promise, combined with the fact that we still don’t understand exactly how they work, has made them one of the most compelling mysteries in modern science.
Their biggest wrinkle is that they need to be cooled to very, very low temperatures to work—close to absolute zero, -460°F. A quarter century ago, scientists discovered a new class of superconductors called cuprates that can work at -280°F—positively sizzling compared to the existing generation. Since then, scientists have been hard at work exploring cuprate behavior in hopes of finding out how they work and of pushing the operating temperature closer to room temperature.
So far, the superconductivity community has documented that cuprates have certain characteristics that seem to set them apart and provide a ‘recipe’ for high-temperature superconductivity: their copper atoms sit in a two-dimensional square lattice, have a spin-½ ion, and “talk” to each other magnetically through a phenomenon known as Heisenberg exchange.
The thought goes that if other materials share these characteristics, they could be undiscovered superconductors—or if they are missing just one or two ingredients, they could provide clues to how cuprate superconductivity works. (Studying systems that are very similar, but with one ingredient missing, is a classic scientific technique).
An international Argonne-led team decided to try their luck with iridium oxides. These, it turns out, have all three characteristics—they are built up of spin-½ ions on a two-dimensional grid structure, and the ions follow the expectations of Heisenberg exchange behavior—but no one has observed superconductivity in them. Yet. The reason is that most cuprates need to have mobile charges in the form of extra electrons or holes added in a process called doping before they become superconducting, and iridates have been very resistant to this process.
B.J. Kim, one of the Argonne authors, thought to add potassium ions to the surface as a way of providing these charges. As he measured the electronic structure, he found that a very thin top layer of the iridate started to behave as though it were doped into a metallic state, the same kind of doping needed for cuprate superconductors. More importantly, Kim found the doped iridate shared a key electronic structure found in the cuprates—a Fermi arc that evolved into a closed Fermi surface as he added more potassium ions or warmed the sample.
Although they did not find evidence for superconductivity, the team plans to continue exploring this material and related iridium oxides.
“The work is an existence proof that these very special Fermi arcs can be found in materials other than cuprates—which could itself suggest that the family of superconductors could be much larger than currently known,” said Argonne scientist John Mitchell, one of the coauthors of the study. “It will also make us look very carefully at how these arcs may or may not result from the same underlying physics as the cuprates.”
The study, titled “Fermi arcs in a doped pseudospin-1/2 Heisenberg antiferromagnet”, first appeared in Science Express on June 12. The other Argonne author on the study was then-postdoctoral researcher Q. Zhao. Samples were prepared at Argonne and analyzed with angle-resolved photoemission spectroscopy at sister lab Lawrence Berkeley National Laboratory’s Advanced Light Source. Other authors on the paper included scientists from Berkeley’s Advanced Light Source, the University of Michigan and the Max Planck Institute for Solid State Research in Germany.