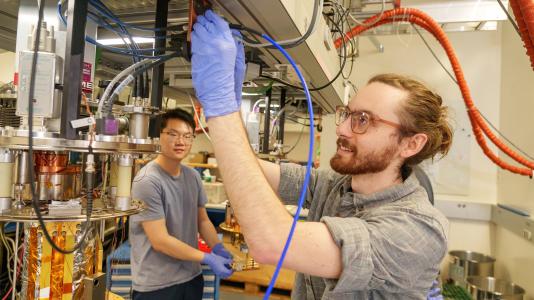
When we listen to our favorite song, what sounds like a continuous wave of music is actually transmitted as tiny packets of quantum particles called phonons.
The laws of quantum mechanics hold that quantum particles are fundamentally indivisible and therefore cannot be split, but researchers at the U.S. Department of Energy’s (DOE) Argonne National Laboratory and the Pritzker School of Molecular Engineering (PME) at the University of Chicago are exploring what happens when you try to split a phonon.
“Those atoms all have to behave coherently together to support what quantum mechanics says they should do. … It’s kind of amazing. The bizarre aspects of quantum mechanics are not limited by size.” — Andrew Cleland, Argonne and UChicago PME
In two experiments – the first of their kinds – a team led by Andrew Cleland, a scientist at Argonne and UChicago PME, used a device called an acoustic beam splitter to “split” phonons and thereby demonstrate their quantum properties. By showing that the beam splitter can be used to both induce a special quantum superposition state for one phonon and further create interference between two phonons, the research team took the first critical steps toward creating a new kind of quantum computer.
The results are published in the journal Science and built on years of breakthrough work on phonons by the team. The work is supported in part by Q-NEXT, a U.S. DOE National Quantum Information Science Research Center led by Argonne.
“Splitting” a phonon into a superposition
In the experiments, researchers used phonons that have roughly a million times higher pitch than can be heard with the human ear. Previously, Cleland and his team figured out how to create and detect single phonons and were the first to entangle two phonons.
To demonstrate these phonons’ quantum capabilities, the team — including Cleland’s graduate student Hong Qiao — created a beam splitter that can split a beam of sound in half, transmitting half and reflecting the other half back to its source. (Beam splitters already exist for light and have been used to demonstrate the quantum capabilities of photons.) The whole system, including two qubits to generate and detect phonons, operates at extremely low temperatures and uses individual surface acoustic wave phonons, which travel on the surface of a material, in this case lithium niobate.
However, quantum physics says a single phonon is indivisible. So when the team sent a single phonon to the beam splitter, instead of splitting, it went into a quantum superposition, a state where the phonon is both reflected and transmitted at the same time. Observing (measuring) the phonon causes this quantum state to collapse into one of the two outputs.
The team found a way to maintain that superposition state by capturing the phonon in two qubits. A qubit is the basic unit of information in quantum computing. Only one qubit actually captures the phonon, but researchers cannot tell which qubit until post-measurement: In other words, the quantum superposition is transferred from the phonon to the two qubits. The researchers measured this two-qubit superposition, yielding “gold-standard proof that the beam splitter is creating a quantum entangled state,” Cleland said.
Showing phonons behave like photons
In the second experiment, the team wanted to show an additional fundamental quantum effect that had first been demonstrated with photons in the 1980s. Now known as the Hong-Ou-Mandel effect, when two identical photons are sent from opposite directions into a beam splitter at the same time, the superposed outputs interfere so that both photons are always found traveling together, in one or the other output directions.
Importantly, the same happened when the team did the experiment with phonons – the superposed output means that only one of the two detector qubits captures phonons, going one way but not the other. Although the qubits have the ability to capture only a single phonon at a time, not two, the qubit placed in the opposite direction never “hears” a phonon, giving proof that both phonons are going in the same direction. This phenomenon is called two-phonon interference.
Getting phonons into these quantum-entangled state is a much bigger leap than doing so with photons. The phonons used here, though indivisible, still require quadrillions of atoms working together in a quantum-mechanical fashion. And if quantum mechanics rules physics at only the tiniest realm, it raises questions of where that realm ends and classical physics begins; this experiment further probes that transition.
“Those atoms all have to behave coherently together to support what quantum mechanics says they should do,” Cleland said. “It’s kind of amazing. The bizarre aspects of quantum mechanics are not limited by size.”
Creating a new linear mechanical quantum computer
The power of quantum computers lies in the “weirdness” of the quantum realm. By harnessing the strange quantum powers of superposition and entanglement, researchers hope to solve previously intractable problems. One approach to doing this is to use photons, in what is called a “linear optical quantum computer.”
A linear mechanical quantum computer – which would use phonons instead of photons — itself could have the ability to compute new kinds of calculations.
“The success of the two-phonon interference experiment is the final piece showing that phonons are equivalent to photons,” Cleland said. “The outcome confirms we have the technology we need to build a linear mechanical quantum computer.”
Unlike photon-based linear optical quantum computing, the UChicago platform directly integrates phonons with qubits. That means phonons could further be part of a hybrid quantum computer that combines the best of linear quantum computers with the power of qubit-based quantum computers.
The next step is to create a logic gate — an essential part of computing — using phonons, on which Cleland and his team are currently conducting research.
Other authors on the paper include É. Dumur, G. Andersson, H. Yan, M.-H. Chou, J. Grebel, C. R. Conner, Y. J. Joshi, J. M. Miller, R. G. Povey, and X. Wu.
This work is supported by the Air Force Office of Scientific Research, Army Research Laboratory, the Department of Energy’s Office of Science National Quantum Information Science Research Centers as part of the Q-NEXT center, and the National Science Foundation.
This story was originally posted by the University of Chicago.
About Q-NEXT
Q-NEXT is a U.S. Department of Energy National Quantum Information Science Research Center led by Argonne National Laboratory. Q-NEXT brings together world-class researchers from national laboratories, universities and U.S. technology companies with the goal of developing the science and technology to control and distribute quantum information. Q-NEXT collaborators and institutions will create two national foundries for quantum materials and devices, develop networks of sensors and secure communications systems, establish simulation and network test beds, and train the next-generation quantum-ready workforce to ensure continued U.S. scientific and economic leadership in this rapidly advancing field. For more information, visit https://q-next.org/.
Argonne National Laboratory seeks solutions to pressing national problems in science and technology. The nation’s first national laboratory, Argonne conducts leading-edge basic and applied scientific research in virtually every scientific discipline. Argonne researchers work closely with researchers from hundreds of companies, universities, and federal, state and municipal agencies to help them solve their specific problems, advance America’s scientific leadership and prepare the nation for a better future. With employees from more than 60 nations, Argonne is managed by UChicago Argonne, LLC for the U.S. Department of Energy’s Office of Science.
The U.S. Department of Energy’s Office of Science is the single largest supporter of basic research in the physical sciences in the United States and is working to address some of the most pressing challenges of our time. For more information, visit https://energy.gov/science.